Will the future of energy be fossil fuels, solar panels, wave power, or nuclear? The merits of each technology are discussed below;
Nuclear
By Oliver Morgan, industrial editorLater this summer, one of the biggest pieces in the complicated jigsaw of future power supply will be put in place with the publication of the government’s energy review. The scope of the review, launched at the end of last year, was intentionally wide. It seeks to set the pattern of the UK’s energy supplies in the coming decades, against the background of trying to reduce carbon emissions by 60 per cent by 2050 and simultaneously ensuring supplies are not disrupted, whether through the intermittence of technologies delivering them, the financial stability of the companies providing them or the reliability of nations supplying sources of power.
However, the intention to appear open-minded was scuppered in May, when Tony Blair declared that nuclear power was back on the energy agenda ‘with a vengeance’ and that it would be a ‘dereliction of duty’ to rule it out. Whichever way it is dressed up, the nuclear question will be the most controversial: there are so many unanswered questions, from the complexities of planning, to the finance question, to dealing with waste. Blair’s predilection is likely only to make it more so.
Government figures show that nuclear currently supplies about 19 per cent of the UK’s electricity. By 2020, this will have fallen to 7 per cent. At the same time, some coal plants will come offline due to a combination of age, European directives and the impact of environmental policies. Although attempts are being made to clean up coal to keep it part of the mix, nuclear supporters such as the Nuclear Industry Association (NIA) argue that atomic energy is indispensable, delivering carbon-free power without the intermittence associated with renewable sources or the threat of interruptions linked with gas.
Their argument was reinforced during the winter, when gas companies hiked prices while Russian giant Gazprom cut supplies to Ukraine, with knock-on effects throughout western Europe. With some estimates indicating that without substantial policy shifts gas could supply up to 80 per cent of UK energy needs by 2020 – with Russia the key provider – the nuclear lobby pressed home the point.
It appears to have done so effectively so far as the Prime Minister is concerned. But there are doubters. His reshuffle has delivered a new Trade and Industry Secretary, Alistair Darling, who is an ally of a more nuclear-sceptic Gordon Brown. However, the energy minister, Malcolm Wicks, who is in charge of the review – a draft of which Blair is said to have seen before making his ‘with a vengeance’ remarks – remains in place.
Wicks has maintained an ‘agnostic’ position in public. But he has addressed some issues which show that he believes there are ways of overcoming the obstacles to investment in atomic power.
The NIA is treading a fine line here – on the one hand arguing that Wicks needs to address these obstacles, while on the other saying new stations do not require public money.
The obstacles can be split into three types: upfront risks involving planning and building reactors on time and to budget; finance, involving the costs of providing electricity throughout the life of stations against the background of volatile prices; and back-end risks to do with disposing of nuclear waste.
The NIA indicates that the government must address upfront risk with a clear planning framework. It points to the six-year process for approving Sizewell B, the last station built in the UK, which opened in 1995. It believes there should be early review of designs, examining their ‘licensability’ and minimising the scope of future inquiries.
On plant construction, the NIA argues that modern reactors, such as French group Areva’s EPR – one of which is currently under construction in Finland – and Westinghouse’s AP1000, can be built for a fraction of former budgets.
Construction costs are sensitive to a number of assumptions – for example, how many stations are built. The NIA uses these arguments to press for a series of plants. It points to a project in South Korea, where the cost of a fifth reactor in a series of newbuilds was only 69 per cent of the first. But licensing ‘off the shelf’ designs to fit local regulations is complicated; economic consultancy Oxera calculates that it can cause 20 per cent cost increases, as well as delays that affect the delivery of forward contracts.
History makes the point. Sizewell B was estimated at about £2bn. It cost nearly £4bn. It was completed late after the largest ever UK public inquiry. Problems were caused by adapting the design from US to UK standards – a process that the Nuclear Installations Inspectorate confirms would be followed with every new station. Electricite de France, potentially a builder of new nuclear stations in the UK, says simply: ‘In the absence of risk mitigation [associated with licensing, planning etc] by government, nuclear will not be built in the current market.’
There are reasons for scepticism about the financing of plants over their lives, too. Even without budget increases and delays, high construction costs and the inability to turn stations off when they are uneconomic – as can be done with fossil fuels – means that nuclear must run and sell at whatever price it can get in the market, which is liberalised in the UK. If revenue falls below operating costs, disaster can follow – as happened to British Energy, which had to be bailed out by the government in 2002.
Prices have increasingly been determined by the gas price, which is currently high, encouraging the view that nuclear is economic. This will not be enough for investors looking at the 40-year lifespan of a plant.
And then there is the issue of nuclear waste. The UK currently has a decommissioning liability of £70bn, which includes burying radioactive fuel and cleaning up facilities. Last year, the responsibility for dealing with the issue was transferred to the Nuclear Decommissioning Authority, a government agency that will appoint contractors to carry out the tasks.
However, the experience of funding these costs corporately is not good. The companies – state or private – that operated facilities were supposed to put aside funds. The amounts they saved were determined by the view of how much the fund would grow (the discount rate), and were therefore quite arbitrary. But they were not enough in the case of publicly owned BNFL or private British Energy. Until April, policy on what to do with the spent fuel was undecided. Then the Committee on Radioactive Waste Management recommended burying it deep underground.
The announcement was seen as vital in paving the way for the government to come down in favour of new stations. However, the committee’s chairman, Professor Gordon MacKerron, said his recommendations, which are being considered by ministers until July, should not be taken as a green light for newbuilds.
In any case, as BNFL and BE show, decommissioning has a nasty habit of costing more than planned. Investors, already wary of construction-phase cost and time overruns and the volatility of energy prices, will want some kind of cap placed on their liabilities. They will not move until ministers have something to tell them.
Solar
By Julian Graff
It’s past six on a spring evening and in David Merrill’s home in Glastonbury, Somerset, the fridge and freezer hum while the 34-inch LCD television, DVD player and surround-sound system entertain the children.
Yet the electricity that drives all this hasn’t cost him a penny: in fact, the power generated by 42 photovoltaic (PV) solar panels on his south-facing roof have earned him credit with his energy supplier on the occasions his house was empty.
‘I like the idea that we are our own power station,’ Merrill says. ‘Around two thirds of our electricity is supplied by solar and wind power, and solar is the main component. Most importantly for me, there are no emissions. It’s clean, silent, renewable energy.’
Nor is Merrill alone. More than 1,000 bus shelters, timetables and other off-grid street furniture in 11 cities around the country are now powered by solar energy. In Britain, an energy-efficient home could be new-built or retro-fitted with solar panels for less than £8,000, which might save up to 32 tonnes of greenhouse gas emissions within its 30-year lifespan, while adding as much as £10,000 to the house’s value. Government grants might halve the outlay, and business clean-energy grants could mean a rebate of up to 60 per cent.
Around the globe, energy generated by the light of the sun and captured by a raft of solar technologies helps to warm, cool and power millions of businesses, community projects and homes.
Solar power seems fittingly to be emerging as a sunrise industry, driven by falling production costs, rising gas and electricity prices, and concerns about global warming and energy security. It is a solution to the problems faced by more than 2 billion people on the planet with no other access to electricity supplies.
Energy research firm Clean Edge calculates that the total solar industry will expand from $11.2bn in 2005 to $51bn in 2015, creating tens of thousands of new jobs. The consultancy and research firm Solarbuzz says the PV sector alone raised more than $1.8bn on capital markets over the past 12 months, and believes that worldwide solar industry revenues will reach $18.6bn to $23.1bn in 2010. From an admittedly tiny base, industry experts say solar electricity demand has grown by 20-25 per cent a year over the past 20 years.
Room for growth and innovation certainly exists: cumulative solar energy production at present accounts for a mere 0.01 per cent of total global primary energy demand and is two to three times more expensive than traditional supplies, although costs are continually falling.
‘Solar energy is still a vast untapped resource,’ says Jeremy Leggett, chief executive of London-based Solarcentury, a leading solar PV consultancy, which supplied the tiles for David Merrill’s roof and which works with architects, house builders and businesses to supply clean, secure energy. ‘In a day, enough energy arrives from the sun to power the whole world for several years,’ Leggett continues. ‘In the cloudy UK we can provide more electricity than the country currently uses – and it’s an electricity-profligate country – just with solar PV on existing rooftops.’
Leggett, an award-winning scientist, former oil industry consultant and Greenpeace campaigner, set up Solarcentury seven years ago. His books, The Carbon War and Half Full, expose the politics and vested energy industry interests helping to fuel global warming and predict that oil production will peak in 2008 – far earlier than previous estimates. This oil shortfall, combined with the accelerating effects of global warming, will mean ‘havoc and ruination for ecosystems and economies’, he says. ‘But out of that can come a pretty big silver lining to the cloud because we get the opportunity to get it right this second time around with alternative energy.’ The 50-strong team at Solarcentury isn’t waiting for the lights to go out. The company has just achieved its first profits (about £500,000) in a year when sales more than doubled to £15m. Last month it concluded a £5.5m private equity financing deal led by the US Silicon Valley firm VantagePoint Venture Partners, including participation from existing investors, Scottish and Southern Energy, together with the conversion of a £1.4m investor loan to equity.
Its profile has been further raised this year by a Department of Trade and Industry Award for solar research in partnership with Reading University, and two building-trade gongs for what is said to be the world’s first fully integrated solar roof – a homegrown and patented combination of solar thermal and solar PV tiles to generate hot water and electricity, even in cloudy conditions.
Solarcentury’s headquarters in Waterloo, south London, was the venue of choice when Prime Minister Tony Blair made his September 2004 speech on the perils of climate change. But Leggett, who serves on the government’s Renewables Advisory Board, was not impressed – ‘he made all sorts of promises that didn’t have much substance to them’ – and his opinion was confirmed by the March 2006 budget, which, in Leggett’s view, actually reduced funding for PV installations to about £5m a year for the next three years. ‘Our competitors [chief among them Japan, Germany and some US states] have support programmes for solar PV measured in billions of pounds, not millions, and stretching over decades. These programmes have the kind of scale and continuity that attract private investors. In the UK we have another drip-feed.’
And while our government appoints a ‘special representative’ on climate change, Germany already boasts a 10MW photovoltaic plant, India has begun building Asia’s largest solar generating facility, and a privately-financed $115m, 18MW PV plant – the world’s largest of its kind – is under construction in Nevada, with its future output already purchased by the US Air Force.
Harnessing the sun’s energy
Concentrating Solar Power: CSP devices optically focus the sun’s thermal energy to drive a generator or engine, using lenses or mirrors arranged in dish, trough or tower configurations. Conventional power stations produce between 500-3,000MW.
Photovoltaic: From the words ‘photo’ meaning light and ‘voltaic’ referring to the production of electricity. PV solar panels are comprised of several connected cells made out of semiconducting materials (usually mono or multi-crystalline silicon or a mix of both) sandwiched between metallic electrodes. When exposed to light, the semiconductors release electrons and produce direct current electricity, which is converted into alternating current for home or business. Silicon solar cells are currently in short supply and relatively expensive. Four firms – two are offshoots of oil giants BP and Shell – account for 50 per cent of PV cell production.
Solar Water/Air Heating: Rooftop panels or wall cladding which absorb radiation to heat air or water for use in a building. Can be ‘open loop’, in which the water to be heated flows through a rooftop collector, or ‘closed loop’, in which the collector is fitted with an antifreeze solution that passes through a heat exchanger. Thin-film technology – think of a solar-powered calculator – is deposited on a base of glass, plastic or metal. Theoretically cheaper to produce, it can be less efficient at converting light to electricity.
Waves
By Robin McKie, science editor
Billia Croo beach is one of the glories of the Orkney islands: a lengthy cove, washed by Atlantic rollers, where seals snooze in the sun and oystercatchers and gannets wheel overhead. On a good day the beauty of the place is breathtaking.
But when the weather turns, as it does with grim frequency in the Orkneys, Billia Croo becomes distinctly inhospitable and, sometimes, terrifying. Hurricane-force winds batter the coast and 40ft waves smash on to the beach. Orcadians make themselves scarce at such times.
But not Neil Kermode, head of the European Marine Energy Centre (Emec) at nearby Stromness. Such meteorological mayhem is his idea of heaven, he says, for it perfectly demonstrates the potential of marine energy.
‘When you look west from Billia Croo, there are several thousand miles of Atlantic and not much else,’ Kermode points out. ‘Waves can build up tremendous energy over that distance. Then it is all dumped on Billia Croo. So if you want to test wave power systems, this is the perfect place. If they survive here, they will survive anywhere.’
Hence the decision in 2002 – by Highland and Islands Enterprise, backed by the Scottish Executive and the Carbon Trust – to establish Emec and its wave energy test bed at Billia Croo. Cables, each thicker than a boxer’s forearm and capable of carrying 2.5MW of power, connect four seabed berths in the bay to a National Grid station on the shore.
‘Our job at Emec is to provide a test bed, support and expertise so wave power developers can try out ideas, carry out trials of their equipment and attempt to supply power to the grid,’ says Kermode. ‘If wave energy is going to happen in Britain, it will happen here first.’
Waves are generated in mid-ocean and are primarily driven by the winds that sweep over the sea’s surface. According to a recent Carbon Trust report, UK marine energy plants – both wave and tidal – could produce 20 per cent of our electricity and replace the country’s ageing atomic reactors as they are closed down over the next 15 years. No need to go nuclear, say enthusiasts: let the might of the sea provide power for our homes.
‘The UK leads the world in marine renewable technology,’ says John Callaghan of the Carbon Trust. ‘Given our superb natural resources and long-standing experience in offshore oil and gas, shipbuilding and power, the UK is in a prime position to accelerate commercial progress in the marine energy sector.’
Already one highly promising device has been tested at Billia Croo: the Pelamis P-750, designed and built by Ocean Power Delivery of Edinburgh. It looks like a floating high-speed train; its undulations in the sea are transformed into power by hydraulic rams that push fluid through a turbine to generate electricity. Now it is to form the core of the world’s first commercial wave power station.
It sounds great; the trouble is that it will be set up not in Britain but off the coast of Portugal, near Povoa de Varzim, where it will provide 2.5MW of electricity – enough for 1,500 homes – to the country’s power grid. In addition, the company has a letter of intent from the Portuguese government for the construction of another 30 machines, provided that the first three work well.
It is good news for Ocean Power Delivery, of course. But the fact that development of this highly promising system is now being pursued by countries other than Britain worries many observers. Like any fledgling device, Pelamis needs support while its bugs are ironed out. That support should be coming from the UK, not Portugal. Then British firms would gain from the feedback.
Officials point out that the government recently set up a £50m fund – the Marine Renewable Deployment Fund – for backing marine energy devices. Most experts say this is simply not enough. ‘Britain’s marine energy industry is going to need at least £200m of support if it is going to get over development problems in devices,’ says Kermode.
Callaghan agrees: ‘The government is effectively offering enough funding for the development of five projects. After that, the cash will run out.We need to provide long-term support and nurture this technology carefully. We lost out over the development of wind turbines; we mustn’t let that happen with marine power. The government has got to commit itself for a significant long-term investment.’
Wave power is only one form of marine energy, of course: there is also tidal power. While waves are generated by winds, tides are caused by the moon dragging a bulge of water round the globe. Exploiting tidal energy involves the development of a very different type of device – in fact, most of the fledgling tidal power generators look a bit like underwater wind turbines. Later this summer, their builders will find out how well they work – when they are put through their paces at Emec.
At a site off the Orkney island of Eday, in the Fall of Warness channel, Emec engineers are building a test bed similar to the one at Billia Croo, but dedicated to tidal devices. ‘The tides off Eday are some of the fiercest in the world,’ says Kermode. ‘The waters rip between the North Sea and the Atlantic, through the middle of the Orkney Islands, past Eday at nearly four metres a second. These are really dangerous waters, but perfect for testing tidal devices.
‘At present, both tidal and wave energy is relatively expensive,’ adds Kermode. ‘However, we expect costs to drop dramatically, just as they have done with wind power. Twenty years ago, we were testing all sorts of different turbine designs. Then we worked out the most effective type and have been slowly reducing costs of construction and operation ever since. That is what should happen with marine power. There is tremendous potential here for generating significant levels of electricity for this country over the next couple of decades.’
Coal
By Oliver Morgan
Clean coal may sound like an oxymoron, but the technology behind it is seen by policymakers, environmental campaigners and industry as a key tool in meeting the world’s growing energy needs while cutting down on harmful carbon dioxide emissions.
Confidence is growing that the technology to burn coal in power stations while cutting emissions by up to 90 per cent is feasible, and that market frameworks can be designed to make it economic in the long term.
Formidable obstacles remain, though. Western governments are not prepared to finance the necessary investment, and countries such as India and China are unwilling to pay more for the energy that will power their economic growth.
With the International Energy Agency predicting that world energy demand will increase by 60 per cent by 2030 and that the current 80 per cent contribution of fossil fuels to global supply will remain constant – implying a 62 per cent rise in emissions – a solution is urgently needed.
The government, which is committed to reducing CO2 emissions by 60 per cent by 2050, considers clean coal technology, known as carbon capture and storage (CCS), as the most promising means of continuing fossil fuel use without its environmental cost. Other alternatives – more efficient combustion or switching to gas – deliver reductions in emissions that are far lower than the 85 per cent of which it considers clean coal capable.
In its carbon abatement technologies (CAT) strategy document, the government says the technologies – which involve separating out carbon dioxide from fuel (coal or gas), compressing it, and storing it underground – are ‘the most radical of the CAT options’. However, it adds that CCS is the least commercially developed of the CAT options because at present ‘there are no policy measures in place to achieve the high levels of CO2 abatement that can be delivered by these technologies… hence no market to pull through to commercial development’.
This does not mean that commercial companies are not experimenting with the technologies, and taking the first steps towards testing the market for them. The oil and gas producer BP, and energy giants Eon, RWE and Scottish and Southern are planning CCS projects in Britain and further afield, each applying different technological solutions.
Jeff Chapman, of the Carbon Capture and Storage Association, an industry body, says: ‘The technologies are now here and we believe it is deliverable.’ He points to the fact that at Sleipner, in the Norwegian sector of the North Sea, 1 million tonnes of CO2 has been injected into underground formations each year since 1996 in a project run by the country’s oil group Statoil.
The technologies split into three types (see below). The first is known as post-combustion capture, which separates CO2 from the flue gas produced after coal is burnt in a conventional power station. In the second, pre-combustion capture, fuel is separated into carbon dioxide and hydrogen, which is then burnt in a gas turbine, while the third, oxyfuel combustion, involves burning fuel in a mixture of oxygen and CO2, which separates the CO2 during the process.
BP, along with Scottish and Southern, is investigating pre-combustion technology for use at an existing power station site at Peterhead in Scotland. The £330m proposal is to build a separation unit and a 350MW turbine to burn the hydrogen. Separated CO2 would be piped into the disused Miller gas field in the North Sea by reversing the flow in existing pipelines.
Meanwhile, Eon, the German energy business that bought the Powergen utility, is examining oxyfuel combustion at its test station, with the aim of using it at a new 450MW, €790m plant in Lincolnshire. RWE, the German company that owns Npower, is considering post-combustion technology for a 1,000MW plant at Tilbury, east London.
Eon’s Derek Farthing says all the technologies are now well understood, but each has pros and cons. ‘Pre-combustion has more engineering challenges but they may be worth overcoming because of the efficiency it generates and the large carbon reduction it can deliver [up to 90 per cent].’ It produces smaller volumes of gas, needing a smaller and cheaper plant.
‘Post combustion scrubbing [the CO2 is “scrubbed” out of the flue gas] is probably the easiest and would be the quickest to develop. It can also be most easily retro-fitted to existing power stations… But the carbon saving is the least of the three. Oxyfuel is highly efficient and clean, but there are technical problems with burning fuel in oxygen because it creates extremely high temperatures.’
The problem, however, is not simply technical. In the case of Britain, the economics do not work yet.
The International Energy Agency points out that market mechanisms to encourage non-carbon energy are either not well enough developed or do not apply to CCS. ‘The European Emissions Trading Scheme [which sets caps on companies for the emissions they are able to produce, but allows them to buy permits if they exceed it – thus generating a price per tonne of carbon] does not fully reflect the cost of climate change.’
The renewables obligation – which obliges energy suppliers to source an increasing percentage of their supply from sources such as wind and biomass power – does not apply to CCS, and environmentalists believe that it should not because it could disrupt development of these technologies.
Chapman believes that a reward system should attach a value to the carbon saved by CCS. ‘If we get a solution to this, the fact that we have fossil fuel power stations can be seen as part of the solution to the global warming problem, rather than its cause.’
Capture and store
Where to store it
Carbon dioxide that is separated is then compressed into a liquid and piped away. There are four main options for long-term storage sites: depleted oil reservoirs; depleted natural gas fields; deep saline aquifers; unmineable coal seams. There are three technologies:
Pre-combustion capture Fuel reacts with oxygen or air to produce carbon monoxide and hydrogen. The CO reacts with steam to make CO2 and more hydrogen. The CO2 is separated and hydrogen is used as fuel in the turbine.
Post-combustion capture CO2 is separated from the flue gas. It is ‘scrubbed’ in an amine solvent, which is then heated to release CO2.
Oxyfuel combustion Fuel is burnt in an oxygen/CO2 mixture to produce flue gas rich in CO2. Oxygen is made in a separate air-separation unit. High temperatures are moderated by recirculating some flue gas into the combustion chamber.
21.03.2007
Four elements in struggle for world power.
Industry News
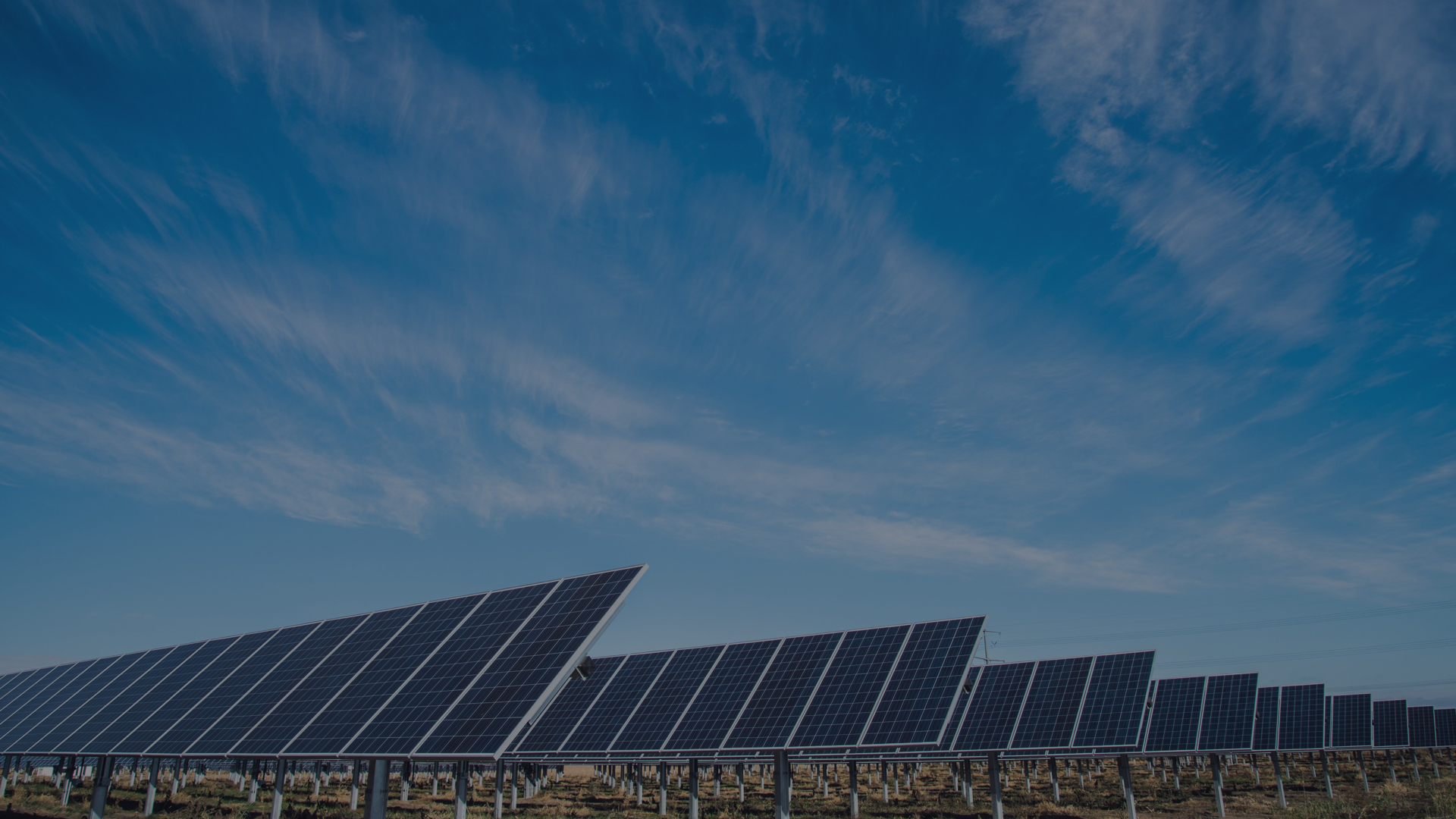